Recently, Professor Li Chaohong's team from the School of Physics and Optoelectronic Engineering and the Institute of Quantum Precision Measurement at Shenzhen University proposed the theory of topological anti-energy bands based on the waveguide quantum electrodynamics system. They revealed topologically dependent photon scattering. The relevant findings were published on September 8, 2023, in the prestigious international physics journal "Physical Review Letters."[https://doi.org/10.1103/PhysRevLett.131.103604].
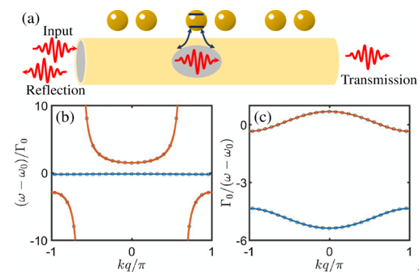
The band theory is a cornerstone of condensed matter physics. Under the influence of periodic lattice potentials, the energy of electrons varies continuously with the lattice momentum, forming energy bands. Energy band theory describes how the energy levels of electrons vary as a function of their momentum in a solid material. The existence of band gaps between these bands determines whether a material behaves as a conductor, semiconductor, or insulator. For a long time, attention was focused on the energies of these bands, while the overall phase of the electronic Bloch states within the bands was often overlooked. It wasn't until Thouless used Bloch states to define topological invariants to explain the integer quantum Hall effect that people began to realize the crucial role played by the topological phase of quantum states. Predictions and discoveries of novel topological quantum states such as topological insulators, topological semimetals, and topological magnetic materials have been made using topological band theory. It has become a powerful tool for studying topological quantum states.
In recent years, researchers have explored how to exploit topological properties to design and manipulate the behavior of light, leading to the gradual development of the emerging field of topological photonics. Early efforts in topological photonics primarily focused on simulating topological models and quantum states from condensed matter physics. Due to the unique properties of photonic systems, they can go beyond simple simulations of topological quantum states. For instance, adding gain to a photonic topological insulator can enable the realization of topological insulator lasers with superior properties such as enhanced monochromaticity, emission efficiency, and stability. Understanding the influence of topological properties on the interaction between light and atoms will pave the way for the development of topologically protected photonic devices, quantum communication, and quantum information processing. The waveguide quantum electrodynamics system, as shown in Figure (a), is a hybrid quantum system where waveguides are coupled to atoms, exhibiting strong interactions between light and atoms. It serves as an ideal platform for studying quantum topological states and photon scattering. However, in the waveguide quantum electrodynamics system, the presence of photon scattering leads to the splitting of energy bands into two discontinuous branches, as depicted in Figure (b), rendering conventional topological band theory inapplicable. There is an urgent need to develop new theories to investigate topological quantum states in waveguide quantum electrodynamics systems.
To address the failure of conventional topological band theory in waveguide quantum electrodynamics systems, Professor Li Chaohong's team first proposed that the reciprocal of energy varies continuously with the lattice momentum, resulting in the formation of continuous inverse bands, as illustrated in Figure (c). They also revealed the relationship between the topological phase in the inverse bands and photon scattering. By calculating the topological Berry phase of the inverse bands, they discovered topological phase transitions determined by the incident photon frequency, contrasting sharply with the topological phase determined by the electronic material structure in condensed matter physics. The team found that the system exhibits flat bands and dark Wannier states supported by these flat bands. Due to their absence of frequency shift, these dark Wannier states possess ultra-long lifetimes and ultra-narrow linewidths, making them suitable for quantum information storage and precise frequency measurement. Despite the absence of topological edge states at boundaries due to radiation decay in waveguide quantum electrodynamics systems, the team discovered a unique bulk-edge correspondence in this system. In topologically nontrivial cases, scale-invariant localized states are distributed across a single inverse band, while in topologically trivial cases, they are distributed across two inverse bands. One of the most surprising findings is that the entanglement number of photon scattering depends not only on the topological phase of one inverse band but also on the parity of the unit cell number. These results reveal the rich and complex topological quantum states in waveguide quantum electrodynamics systems, inspiring further research into topological behaviors in the interaction between light and matter.
This achievement, titled "Topological Inverse Band Theory in Waveguide Quantum Electrodynamics," was published in "Physical Review Letters." Shenzhen University served as the first and corresponding institution, with Associate Professor Ke Yongguan from Sun Yat-sen University as the first author, Professor Li Chaohong from Shenzhen University, and Professor Yuri Kivshar from the Australian National University (honorary professor at Shenzhen University) as co-corresponding authors. This research was supported by the National Key Research and Development Program, the National Natural Science Foundation of China, and key research and development projects in Guangdong Province. Since joining Shenzhen University in 2022, Professor Li Chaohong's team has focused on research directions such as quantum engineering and artificial quantum systems, quantum correlations and novel quantum states, and quantum precision measurement and quantum sensor devices. Leveraging the School of Physics and Optoelectronic Engineering, they established the "Shenzhen University Institute of Quantum Precision Measurement" and participated as one of the co-building units in the construction of the "Guangdong-Hong Kong-Macao Greater Bay Area Quantum Science Center," making commendable progress in innovative research and talent introduction. Additionally, it is worth mentioning that the prediction made by Professor Li Chaohong's team in 2017 regarding bound-state topological transport and topological resonant tunneling (with the first author being Ke Yongguan at the time of publication—a doctoral student at Sun Yat-sen University) was experimentally verified by Professor Esslinger's group at ETH Zurich, with their experimental results published in "Nature Physics" [https://doi.org/10.1038/s41567-023-02145-w], and we were invited to write a review for "Nature Physics" [https://doi.org/10.1038/s41567-023-02169-2].